DNA sequencing was revolutionized after scientists discovered a new bacterium in the hot springs of Yellowstone National Park, and today it’s a common research technique.
This game-changing bacterium is called Thermus aquaticus, and it can withstand temperatures as high as 80°C. It’s an example of an extremophile – an organism capable of living in extreme environments such as those with very hot or very cold temperatures, high levels of salinity or acidity, a high ambient pressure or ionizing radiation.
“Extremophiles have developed a range of strategies for surviving or even thriving in hostile environments,” says Ianina Altshuler, a tenure-track assistant professor at EPFL’s Microbiome Adaptation to the Changing Environment (MACE) laboratory. Her research group is studying extremophiles living in cryo-environments to understand how they’ve adapted to ultralow temperatures. “Many of these organisms produce proteins suited specifically to the cold, such as enzymes whose optimal activity temperature is relatively low,” says Altshuler.
Famous for their survival strategies
The exceptional capabilities of extremophiles have made the headlines in some cases. For instance, Deinococcus radiodurans, a bacterium discovered in canned food in 1956, can withstand high doses of gamma radiation. Halomonas titanicae, a bacterium isolated from the wreckage of the Titanic in 1991, can live in highly saline environments; its presence accelerates the corrosion of metal – to the point where the wreckage’s metal parts could deteriorate completely.
Thermus aquaticus was discovered by Thomas D. Brock and Hudson Freeze in Yellowstone National Park in 1969. The bacterium is known for the fact that it creates a protein called Taq polymerase– a heat-resistant enzyme that’s active at unusually high temperatures: 70° to 80°C.
Heat-resistant enzyme
That discovery led to the invention of the polymerase chain reaction, or PCR (as in the PCR tests we took during the pandemic), which enables scientists to select specific DNA fragments and then amplify them by making a large number of copies. “We can’t generally observe DNA fragments without amplifying them – they’re too small,” says Altshuler. The PCR method, by enabling such amplification, paved the way for DNA sequencing to become a common practice in research labs. The method made it possible to map the human genome. It’s also used to make medical diagnoses, spot genetic mutations and support research in forensics, agriculture, environmental science and so on. It also earned its inventor, Kary B. Mullis, the Nobel Prize in Chemistry in 1993. Before PCR, the process of DNA sequencing was very lengthy and quite expensive.
How did the discovery of the Taq polymerase result in this important research technique? To amplify a DNA fragment, scientists need to expose it to a series of thermal cycles: first the fragment is heated, causing it to open up, then the temperature is lowered so that an enzyme can copy the strand before it closes again, and the cycle is repeated. While most common enzymes are active at around 30°C – which is too low for these thermal cycles – the Taq polymerase can withstand the higher temperatures that are required.
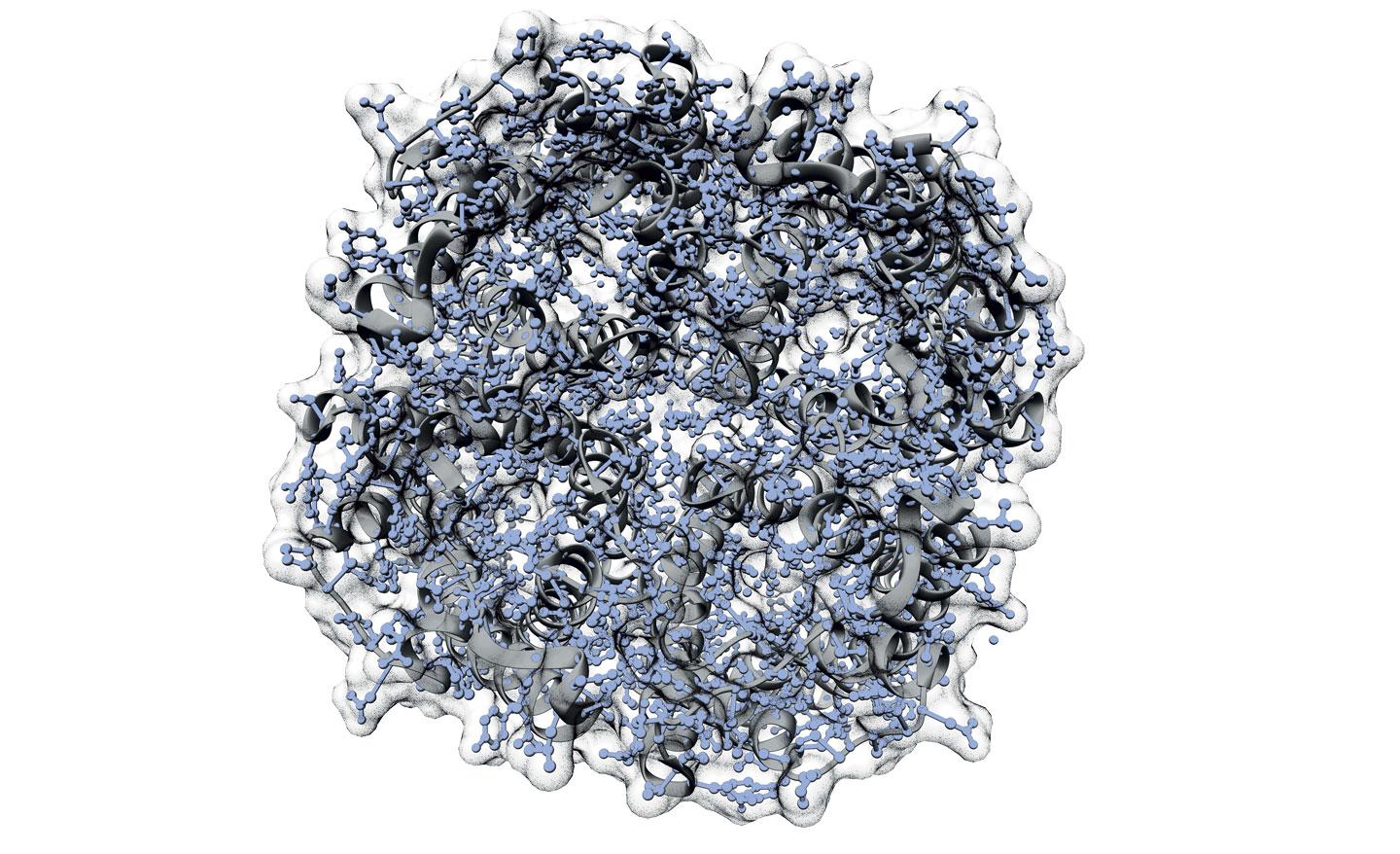
From the Latin aqua meaning water and poros meaning passageway, aquaporins are proteins that allow water molecules into or out of cells on a very selective basis. Found in all living organisms – from bacteria and yeast to plants and animals – they have an hourglass shape through which only water molecules can pass, one by one. Owing to their exceptional filtering and permeability properties, aquaporins have a number of applications in health care, improving dialysis, for instance, and in chemical engineering for sea water desalination. Aquaporins are also one of the treatment targets for glaucoma, a disease that affects the aqueous humor of eyeballs. Their discovery earned US biologist Peter Agre the 2003 Nobel Prize in Chemistry.
(CC Int’l 4.0)
Comments